The browser you are using is not supported. Please consider using a modern browser.
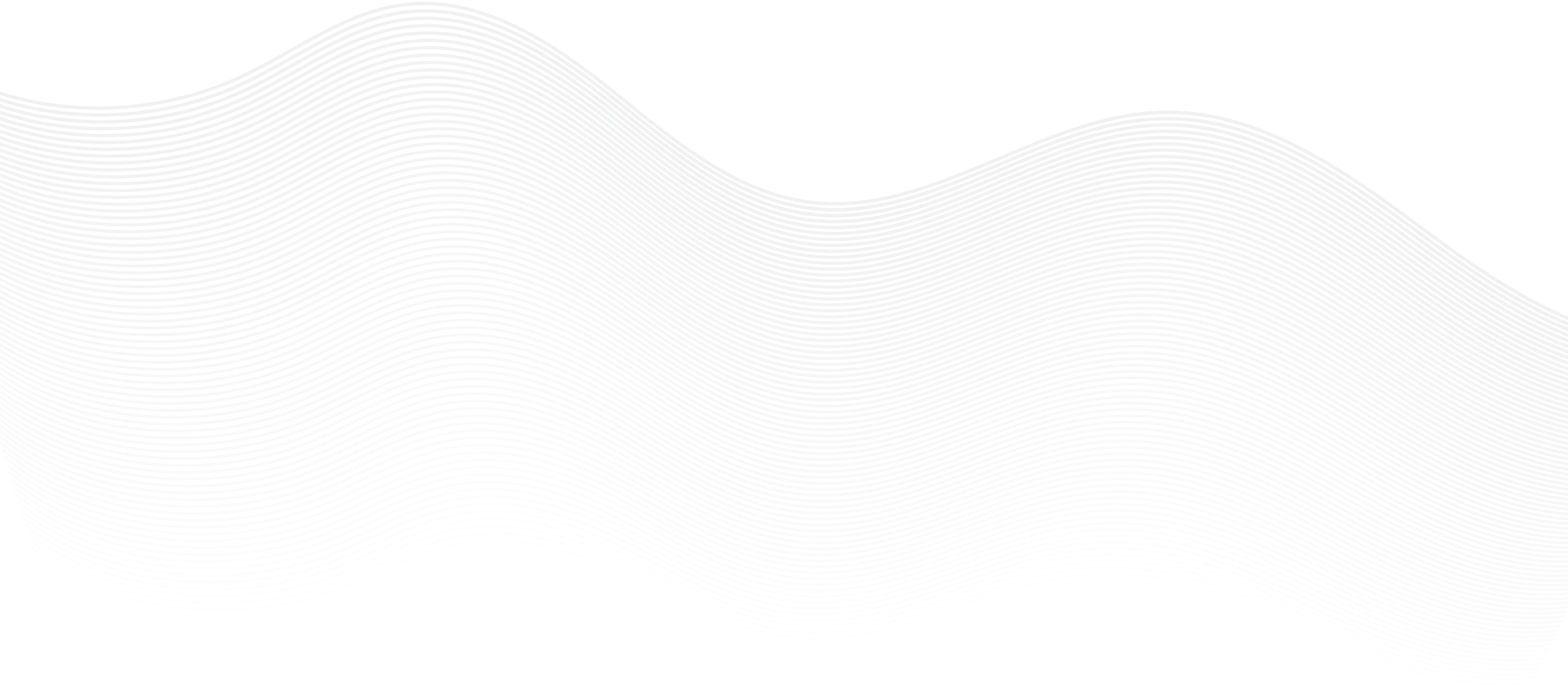
A Powerful New Thermoelectric Material
The science of thermoelectrics has become an increasingly popular field of study in recent years. As the Internet of Things (IoT) and the subsequent demand for small-scale electricity generators grows, so does the need for new, innovative materials and devices to convert heat into electric energy. Scientists at Vienna’s TU Wien have succeeded in the development of a new material that may unlock the door to a new type of energy. The results, presented in the scientific journal Nature, have served as a source of excitement for the scientific community, and an opportunity to further explore the vast potential behind thermoelectrics and their ability to bring big changes to the power industry, as a whole.
The Science Behind the Seebeck Effect
The new material is already breaking records in regard to small-scope energy. Its potential can be attributed to the Seebeck Effect, which states that the temperature difference between two ends of a material can be used to generate electrical voltage. The material, which consists of a thin layer of iron, tungsten, vanadium and aluminum applied to a silicon crystal, was measured at an impressive 5-6 ZT. To put this into perspective, the best thermoelectrics currently known are measured to hold ZT values between 2.5 and 2.8. The new material is anticipated to be effective enough to provide energy for small computer processors and sensors, which will grow in demand in years to come.
There are a number of factors that make the newly discovered material unique, even by thermoelectric standards. One factor is its silicon composition, which changes its structure radically. According to Professor Ernst Bauer of TU Wien, a good thermoelectric material, in addition to demonstrating a strong Seebeck effect, must meet two crucial requirements, including conducting electricity as well as possible, and transporting heat as poorly as possible. Given that thermal conductivity and electrical conductivity are so closely related, materials that meet all requirements are a rarity, which makes the discovery all the more exciting. But how does it work?
While, according to Professor Bauer, the atoms in this material are typically arranged in a strictly regular pattern, a “face-centered cubic lattice”, the addition of a silicon component arranges them in a “space-centered structure”, allowing for a completely randomized atomic distribution. This mixture of regularity and irregularity of the atoms’ arrangement alters the electronic structure of the material, which allows electrical charge to navigate the material in a unique way. The portions of charge that travel through this new material, which are called Weyl Fermions, are responsible for the low electrical resistance achieved.
A Power Discovery Years in the Making
Professor Bauer’s progress in thermoelectrics is the result of years of careful research and study. In 2013, Ernst Bauer established the Christian Doppler Laboratory for Thermoelectricity at TU Wien, where researchers have conducted a variety of thermoelectric studies over the past few years.
While this new material, due to its ultra-thin composition, won’t be able to generate a large amount of energy, it carries other advantages. Being both compact and adaptable, it is thought to be particularly useful for providing energy for small objects, such as sensors and small electronic applications, for which the demand is growing at a fast rate. In an era where the Internet of Things calls for efficient linking of devices, small-scale power will be increasingly useful.